Alexia I. Blake | Alejandro G. Marangoni*
Over the last 20 years, consumers have developed a love-hate relationship with fat. While fat is responsible for the textures and tastes of our favourite foods, such as ice cream, butter, and baked goods, its prevalence in today’s energy-dense diets has caused a drastic increase in obesity rates and cardiovascular disease observed worldwide1-3. Scientific evidence has shown that trans fats have particularly detrimental health effects, as they raise low density lipoprotein serum levels (i.e. bad cholesterol), and decrease high density lipoprotein serum levels (i.e. good cholesterol), which increases the risk of developing metabolic and cardiovascular diseases4-8.
Small amounts of trans fats are naturally present in animal and dairy products, such as milk, meat, and cheese9. Trans fats can also be industrially produced during a process known as hydrogenation10. During this process, hydrogen gas is added to oil, to convert it from a viscous and non-elastic liquid at room temperature into a viscoelastic semi-solid fat. Hydrogenated oils are added to snack foods (crackers, microwaveable popcorn, pizza), baked goods (cookies, donuts, cakes, pies), refrigerated dough, vegetable shortening, margarines, and stick spreads, to enhance their taste, texture, and shelf-life11.

Denmark was the first to respond to the diet-related health crisis in 2003 by restricting the amount of trans fat present in fats and oil to 2% (w/w) (Danish Order N. 160, March 2003). A decade later, the United States’ Food and Drug Administration (FDA) released a preliminary determination that partially hydrogenated oils (PHOs), which are the main dietary source of artificial trans fats, should no longer be labeled as “generally recognized as safe” (GRAS)12. Without GRAS status, food manufacturers must seek approval for their use as food additives.
Canada has been much slower to adopt a stance on the issue, despite recommendations from the Trans Fat Task Force (TFTF), a collaborative initiative between Health Canada and the Heart and Stroke Foundation, to regulate the use of trans fats in foods9. In 2007, Minister of Health, Tony Clement, threatened food manufacturers with regulation if trans fats were not reduced to 2% of the total fat content of vegetable oils and spreadable margarines, and to 5% of the total fat content of other foods available for purchase within two years, as recommended by the TFTF based on the World Health Organization’s recommendation that average daily trans fat consumption for all age groups does not exceed 1% of energy intake1,9. The Trans Fat Monitoring Program revealed in their final report, published in December 2009, that many food products still contained dangerous levels of trans fats, such as cookies, desserts, bakery products, and french fries13. Despite this failure, legislation was not introduced.

Fortunately, under the pressure of domestic legislation and evolving consumer demands, some food manufacturers have already begun to reformulate their products. Simply removing PHOs is not an option due to the contribution that fat provides to the structure, taste, texture, and mouthfeel of the product. Thus, food manufacturers must replace these fats with a material that mimics the behaviour of fat so that the quality of the food product does not suffer.
In Search of Fat Alternatives
Fat is a surprisingly complex system that is difficult to mimic. Despite appearing solid to the naked eye, fat actually contains a considerable amount of liquid oil. This oil is entrapped by a network of fat crystal clusters14, 15. The clusters are aggregates of fat crystal nanoplatelets (CNPs) that are composed of fat molecules, also known as triacylglycerol (TAG) molecules, that stack tail to tail into a lamellar structure.
Depending on the solid fat content, crystal shape, size, and distribution, the functional properties of the fat (melting behaviour, texture, spreadability, flavour) will change15-17. This tunable behaviour is difficult to replicate, and complicates the development of equally versatile and functional replacements.

One strategy involves replacing PHOs with other hardstock fats, such as palm oil, palm kernel oil, and coconut oil18. This has created controversy for several reasons. First, these fats contain very high amounts of saturated fat, which has disputed effects on cardiovascular health4, 19-21. Secondly, the production of these tropical fats has devastating environmental effects, which will only worsen if demand increases22-26. Using locally available oils would result in environmentally-friendly sustainable practices, while also promoting the growth of the Canadian agriculture industry.
A more recent and novel strategy involves replacing the fat crystal network with another network that also structures liquid oil into a semi-solid material that resembles fat. Liquid oil is a more nutritious alternative since it contains very low to zero levels of trans fatty acids, and low amounts of saturates compared to solid fat. In addition, gelled oil may contain high levels of mono-unsaturated and poly-unsaturated oils, which studies have shown to have many positive health benefits5,7,9,20. Moreover, “locally-grown” oils can be used, such as canola oil or soybean oil. Oil structuring can be achieved using two main mechanisms, discussed below.
Oleogels
The first method of structuring oil is gelation. This is achieved by adding one of several gelator species to oil at concentrations below 4% (w/w)17, 27-29. The oil-gelator mix is heated to above the melting temperature of the gelator to disperse the gelator molecules. Upon cooling, these molecules crystallize and form a three-dimensional network that entraps the oil, producing an oleogel. These gels exhibit solid-like behaviour despite containing at least 96% (w/w) liquid oil. Apart from displaying tunable solid-like behaviour that is similar to fat, gelators must also be food grade, be priced realistically, and be available in relatively high amounts. These species can be classified as small molecular or polymer gelators.
Small Molecule Gelators
The building blocks of these oleogels are low molecular-weight organogelators (LMOGs). They self-assemble during crystallization into a three-dimensional network that entraps liquid oil. If LMOGs assemble into a crystalline fiber, their network is referred to as a self-assembled fibrillar network (SAFiN).

Bot and Floter reported that mixtures of phytosterols and oryzanol form SAFiNS in oil29-31. Phytosterols are present in plant membranes at low concentrations (< 1 %), and improve cardiovascular health by lowering low-density lipoprotein serum levels32. Oryzanol is a sterol derivative formed by interesterifying sterols with vegetable oil31. Of the many sterol derivatives, including dihydrocholesterol, cholestanol, and stigmasterol, mixtures of β-sitosterol and γ-oryzanol are the most efficient oil structuring species. However, gelation only occurs if both components are present.
When combined, these molecules self-assemble into tubules that are approximately 7 nm in diameter27, 31. At concentrations between 2-4% (w/w), tubules aggregate into a three-dimensional network that entraps the oil and produces an oleogel. The gels are translucent, meaning that the tubules are smaller than the wavelength of visible light. Gels prepared with a 1:1 molar ratio of the two species exhibit the highest melting temperature and firmness. Changing the molar ratios, the type of sterol, and the type of oil used during esterification to produce sterol esters, alters the mechanical and thermal properties of the gel.
Another group of small molecule gelators are plant-based waxes33-36. Rice bran (RBX), sunflower (SFX), candelilla (CLX), and carnauba (CRX) wax have received a considerable amount of attention over recent years due to their ability to gel liquid oil at concentrations as low as 1% (w/w). Since such a small amount of wax is required to achieve gelation, coupled with the fact that waxes such as RBX and SFX are abundant in nature and commercially available, the addition of wax should be inexpensive and impart little (if any) taste on the final food product. Waxes are already used in a variety of consumer products, including cosmetics, candy coatings, lubricants, and greases37.
Rather than forming a three-dimensional and continuous network like β-sitosterol and γ-oryzanol mixtures, waxes form dispersions in oil. Their platelet-like crystals, ranging between 3-30 µm in diameter, are evenly distributed throughout the oil38. It has been suggested that these crystals gel up to 99% (w/w) liquid oil by entrapping it within the pores of the network, and by adsorbing the oil onto the surfaces of the crystals33, 34, 38.
Like fat, wax oleogels are extremely versatile. Their firmness, melting behaviour, and oil binding capacity can be modified by changing the type and concentration of wax, and by altering the shape and size of the crystals using external processing conditions such as shear and non-isothermal cooling34, 35, 39-41. This flexibility is essential for adapting the functional properties of wax oleogels to be compatible with different food products.
Polymer Gelators
Dey et al. and Laredo et al. were the first to report the gelation of oil using ethyl cellulose (EC), which to date remains the only polymer capable of this feat42, 43. Gelation is achieved by heating > 4% (w/w) EC in oil to the glass transition temperature of the polymer (130 °C), causing polymer strands unfold and become mobile44, 45. During cooling, EC chains interact with other chains via hydrogen bonds to form a cross-linked network that entraps the surrounding oil phase27, 45.
The mechanical properties of EC oleogels depend on the concentration and molecular weight of EC, and the type of oil used44, 45. Increasing the concentration or the molecular weight of the polymer produces firmer gels. The addition of surfactants has a plasticizing effect that lowers the glass transition temperature, meaning that gels can be prepared at lower temperatures. This prevents oil oxidation, which has been shown to increase gel firmness46.
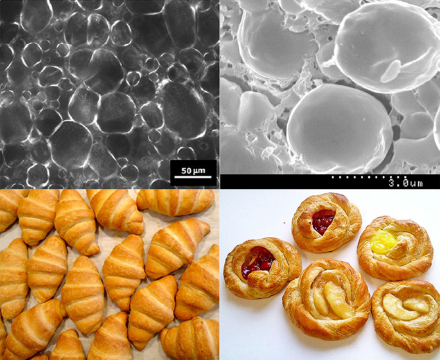
EC oleogels have been evaluated as fat replacements for frankfurter products, which often contain more than 20% fat47. Zetzl et al. reported that there was no difference in the chewiness or hardness of frankfurters made with canola oil EC oleogels compared to control frankfurter made with beef fat45. When only canola oil replaced the solid fat in frankfurter products, oil phase separation and undesirable changes in product chewiness and texture occurred, demonstrating the importance of structuring the oil47. Within the realm of confectionary products, EC has shown to provide heat resistance to chocolate48.
The use of EC as a food additive is already permitted in Europe (Directive 2006/52/EC), but not in North America. This is unfortunate given the efficacy of this polymer as an oil binding agent. The ability to modify its mechanical properties makes it a very versatile oleogel that is likely compatible with a broad range of food applications, and thus a good fat alternative candidate. Hopefully, its use as a food additive in Europe will demonstrate both its safety and effectiveness to the FDA (and Health Canada), leading them to accept Dow Wolff Cellulosics application for GRAS status (GRN. No. 470).
Structured Emulsions
The second method of structuring liquid oil is by incorporating it into an emulsion. Emulsions contain at least two phases that are immiscible with each other, with one of the phases being dispersed as small droplets throughout the other49. Examples include mayonnaise, milk, and ice cream. Compared to traditional emulsions, a structured emulsion displays stronger solid-like behaviour that can be controlled by modifying the ratio of ingredients. One example are oil-in-water (o/w) emulsions that are structured by a hydrated monoglyceride bilayer.
Monoglycerides are among the most commonly used emulsifiers in the food industry, and are known to self-assemble into various configurations in both aqueous and non-aqueous mediums50. In this particular system, monoglyceride molecules self-assemble around oil droplets that are dispersed throughout a continuous aqueous phase and sandwich large volumes of water between layers51-53. The hydrated monoglyceride bilayers are stabilized by hydrogen bonding between adjacent monoglyceride molecules and by electrostatic repulsion between anionic co-surfactant molecules. During cooling, the monoglyceride chains crystallize and encapsulate oil droplets ranging between 1-6 µm in diameter. The final emulsion is a solid at room temperature, even though the monoglyceride is the only solid component and is present at concentrations between 3-6 % (w/w).
This structured emulsion is a commercially available shortening alternative branded as Coasun™. This product is a healthier alternative to baking shortenings, as it contains zero trans and only 7-8 % saturates when the oil phase is comprised of canola oil54. Furthermore, the high water content of these systems, ranging between 30-40 % (w/w), reduces the caloric content by almost 40 % compared to other commercial shortening products55. Added benefits include the ability to encapsulate functional nutraceuticals (phytosterols or β-carotene), and the ability to tailor the nutritional profile of the oil phase by using poly-unsaturated and mono-unsaturated oils52.
Coasun can be modified to act as a roll-in shortening replacement for puff pastry products. Roll-in shortenings are much harder and elastic compared to Coasun56, 57. However, by gelling the oil phase via the addition of wax, or by increasing the monoglyceride content, the mechanical properties of the emulsion can be adjusted to match those of a roll-in shortening.
With any emulsion system, there are concerns about stability and phase separation. The high water content of Coasun is indeed problematic in this respect, as condensation occurs during long-term storage or transportation under warmer temperatures, which may result in microbial growth. This can be mitigated by adding an antimicrobial agent, such as potassium sorbate, or by keeping this product at refrigeration temperatures for long term storage.
Concluding Remarks
Food manufacturers are beginning to limit the use of hydrogenated oils in food in response to changing consumer demands and legislation brought about by the known adverse health effects of trans fats. This has created a need for zero-trans fat replacements. The ability to structure edible oils into functional fat replacements that are free of trans fats, low in saturates, and also contain nutritionally beneficial or locally available oils is a very promising solution to this conundrum that, if adopted by food manufacturers, will lead to the development of healthier manufactured foods.
Figure 1. The Fat Structural Hierarchy. TAG molecules self-assemble into crystalline lamellae that aggregate into nanoplatelets, which further aggregate into larger crystals (spherulites).14
Figure 2. Brightfield light micrographs of wax oleogels consisting of canola oil and (A) 1 % (w/w) rice bran wax, (B) 1% (w/w) sunflower wax, (C) 2% (w/w) candelilla wax, and (D) 4 % (w/w) carnauba wax.
Figure 3. EC oleogels (left) and the polymer network (right) of a soybean oil organogel prepared with cP 45 ethyl cellulose. Oil has been removed by washing the gel with 2 mL of isobutanol45.
Figure 4. Micrographs of cooked comminuted meat batter containing (A) beef fat, (B) canola oil EC oleogels, and (C) canola oil. The white circular globules are fat globules (note: removed during the paraffin embedding process), while the dark surrounding area is the protein network in which some small intact muscle fibers still exist. The scale bar is 100um45.
Figure 5. Optical light micrograph (top left) and cryogenic scanning electron micrograph (top right) of Coasun. When wax is added to the oil phase, Coasun can be used as a roll-in fat replacement for croissants (bottom left) and danishes (bottom right).
Alexia I. Blake and Alejandro G. Marangoni*
Dept. of Food Science, University of Guelph
Guelph, ON N1G2W1
(*Corresponding author email: amarango@uoguelph.ca)
References
[1]
World Health Organization. (2003). WHO Technical Report Series: Diet, Nutrition and the Prevention of Chronic Diseases.
http://whqlibdoc.who.int/trs/who_trs_916.pdf
[2] James, W.P.T. (2008). WHO recognition of the global obesity epidemic. International Journal of Obesity, 32. S120-126.
[3]
World Health Organization. (2015). Obesity and Overweight.
http://www.who.int/mediacentre/factsheets/fs311/en/. Accessed 16 Apr 2015
[4] Aro, A. et al. (1996). American Journal of Clinical Nutrition, 65:1419-1426
[5] Uauy, R. et al. (2009). European Journal of Clinical Nutrition, 63:S68–S75.
[6]
Mensink, R.P., Katan, M.B. (1990). New England Journal of Medicine,
323:439–445.
[7]
Mensink, R.P. et al. (2003). American Journal of Clinical Nutrition,
77(5):1146–1155.
[8] Judd, T. et al. (1994). American Journal of Clinical Nutrition, 59:861–868.
[9] Trans Fat Task Force. (2013). TRANS forming the food supply – Report of the Trans Fat Task Force. http://www.hc-sc.gc.ca/fn-an/nutrition/gras-trans-fats/tf-ge/tf-gt_rep-rap-eng.php
[10] Frazier, R.A. (2009). Chapter 2: Food Chemistry. In: Campbell-Platt, G. Food Science and Technology, Wiley-Blackwell, p 22.
[11]
Food and Drug Administration. (2013). Consumer Updates.
http://www.fda.gov/ForConsumers/ConsumerUpdates/ucm372915.htm
[12] Food and Drug Administration. (2013). Tentative Determination Regarding Partially Hydrogenated Oils; Request for Comments and for Scientific Data and Information. https://www.federalregister.gov/articles/2013/11/08/2013-26854/tentative-determination-regarding-partially-hydrogenated-oils-request-for-comments-and-for.
[13]
Trans Fat Monitoring. (2009). Fourth Set of Monitoring Data.
http://www.hc-sc.gc.ca/fn-an/nutrition/gras-trans-fats/tfa-age_four-data_quatr-donn-eng.php
[14] Marangoni, A.G. et al. (2012). Soft Matter, 8:1275-1230.
[15] Narine, S.S., Marangoni, A.G. (1999). Food Research International, 32:227–248.
[16] Marangoni, A.G., Rousseau, D. (1996). Journal of the American Oil Chemists’ Society, 73:991–994.
[17] Rogers, M. (2009). Food Research International, 42:747–753.
[18] Idris, N. A., Miskandar, M.S. (2007). European Journal of Lipid Science and Technology, 109:422–432.
[19] Ghotra, B.S. et al. (2002). Food Research International, 35:1015–1048.
[20] Mensink, R.P., Katan, M.B. (1992). Arteriosclerosis, Thrombosis, and Vascular Biology, 12:911-919.
[21] Keys, A. et al. (1965). Metabolism, 14(7):776–787.
[22] Brown, E., Jacobson, M.F. (2005). Center for Science in the Public Interest.
[23] Massey, N. (2012). Scientific American. http://www.scientificamerican.com/article/palm-oil-set-to-grow-indonesia-climate-changing-emissions/
[24]
WWF Global. (2004). Palm Oil and Air Pollution.
http://wwf.panda.org/what_we_do/footprint/agriculture/palm_oil/environmental_impacts/air_pollution/.
[25]
WWF Global. (2004). Palm Oil and Climate Change.
http://wwf.panda.org/what_we_do/footprint/agriculture/palm_oil/environmental_impacts/climate_change/.
[26]
WWF Global. (2004). Palm Oil and Forest Conversion.
http://wwf.panda.org/what_we_do/footprint/agriculture/palm_oil/environmental_impacts/forest_conversion/.
[27] Co, E., Marangoni, A.G. (2012). Journal of the American Oil Chemists’ Society, 89:749–780.
[28]
Hughes, N.E. et al. (2009). Trends in Food Science and Technology,
20:470–480. 29.
[29] Pernetti, M. et al. (2007). Current Opinion in Colloid and Interface Science, 12:221–231.
[30] Bot, A., Agterof, W. (2006). Journal of the American Oil Chemists’ Society, 83:513–521.
[31] Floter, E., Bot, A. (2011). Edible Oil Organogels Based on Self-assembled β-sitosterol + γ-oryzanol Tubules. In: Marangoni, A.G., Garti, N. Edible Oleogels: Structure and Health Implications, 1st Edition, AOCS Press, Urbana, pp. 49–79.
[32] Katan, M. et al. (2003). Mayo Clinic Proceedings, 78:965–978.
[33] Dassanayake, L.S.K. et al. (2009). Journal of the American Oil Chemists’ Society, 86:1163–1173.
[34] Blake, A.I. et al. (2014). Journal of the American Oil Chemists’ Society, 91:885–903.
[35] Hwang, H.S. (2011). Journal of the American Oil Chemists’ Society, 89:639–647.
[36] Toro-Vazquez , J.F. et al. (2007). Journal of the American Oil Chemists’ Society, 84:989–1000.
[37] Miyazaki, Y., Marangoni, A.G. (2014). Mater Res Express 1:025101. Material Research Express, 1:025101
[38] Blake, A., Marangoni, A.G. (2015). Food Structure, 3:30-34.
[39] Dassanayake, L.S.K. et al. (2012). Journal of Oleo Science, 61:1–9.
[40] Chopin-Doroteo, M. et al. (2011). Food Biophysics, 6:359–376.
[41] Alvarez-Mitre, F.M. et al. (2012). Food Research International, 49:580–587.
[42]. Laredo, T. et al. (2011). Soft Matter, 7:2734-2743.
[43] Dey, T. et al. (2011). Ethylcellulose Oleogels. In: Marangoni, A.G., Garti, N. Edible Oleogels: Structure and Health Implications, 1st Edition, AOCS Press, Urbana, pp. 295–312.
[44] Gravelle, A.J., et al. (2012). Food Research International, 48:578–583.
[45] Zetzl, A.K. et al. (2012). Food Function, 3:327–37.
[46] Gravelle, A.J. et al. (2013). Food Function, 4:153–61.
[47] Youssef, M.K., Barbut, S. (2009). Meat Science, 82:228–233.
[48] Stortz, T.A., Marangoni, A.G. Food Research International, 51(2):797-803.
[49] McClements DJ (2005) Food Emulsions: Principles, Practices, and Techniques, 2nd ed. 1–5.
[50] Sagalowicz, L. et al. (2006). Trends in Food Science and Technology, 17:204–214.
[51] Batte, H.D. et al. (2007). Food Biophysics, 2:29–37.
[52] Zetzl, A.K., Marangoni, A.G. (2011). Novel Strategies for Nanostructuring Liquid Oils into Functional Fats. In: Marangoni, A.G., Garti, N. Edible Oleogels: Structure and Health Implications, 1st Edition, AOCS Press, Urbana, pp. 19–47.
[53] Batte, H.D. et al. (2007). Food Research International, 40:982–988.
[54]
Coasun: Coasun Advantage – Health Benefits.
http://www.coasun.com/coasun-advantage/health-benefits/
(Accessed 10 Apr 2014).
[55] Coasun: Coasun Products – Food. http://www.coasun.com/coasun-products/coasun-products-foods/ (Accessed 10 Apr 2014).
[56] DeMan, L. et al. (1991). Journal of the American Oil Chemists’ Society, 68:63–69.
[57] Patient, D. (2006). Nutrition and Food Science, 94:33–35.